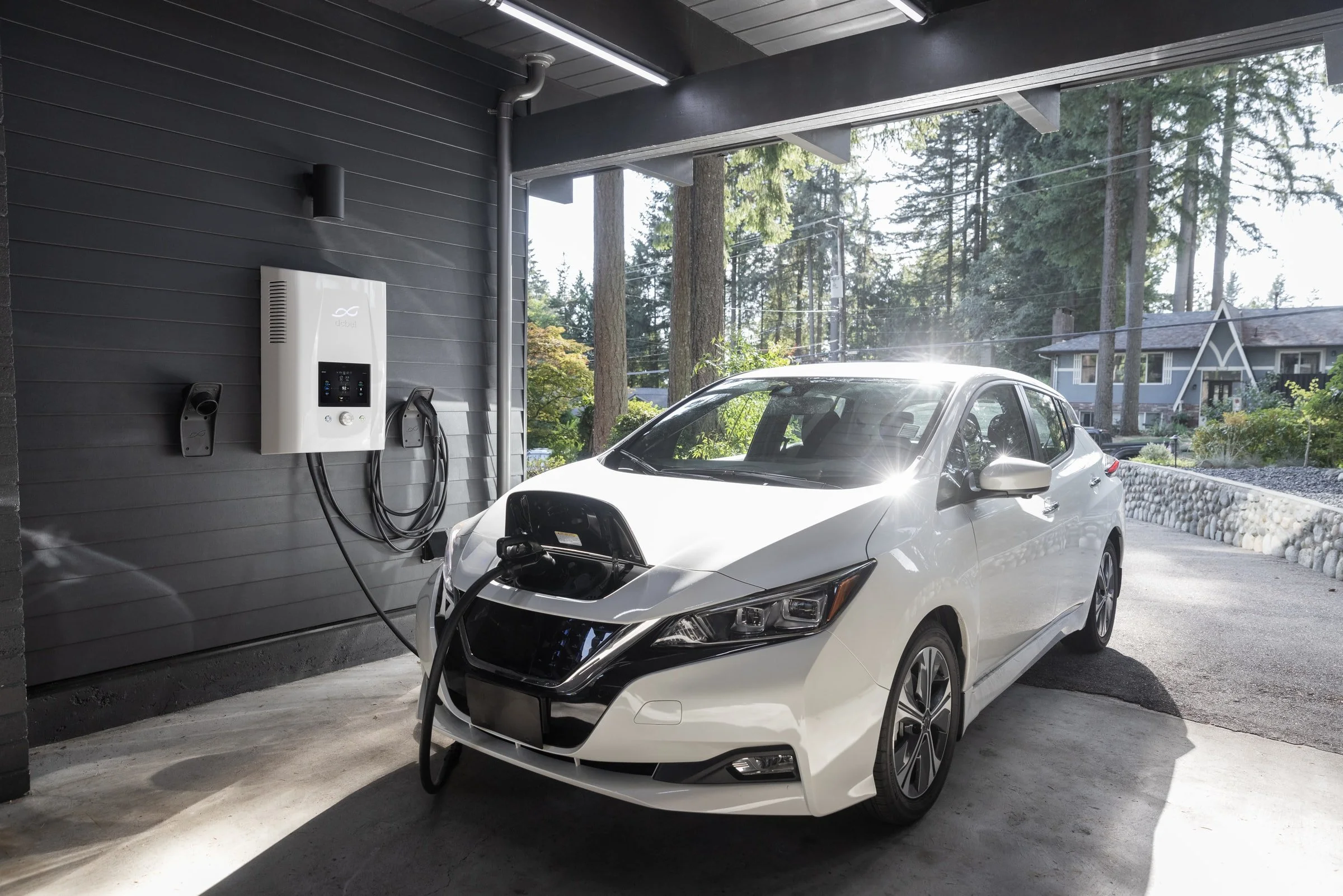
“As reliability concerns grow in parallel with frequent temporary service disconnections (e.g. public safety power shutoffs; PSPS), distributed energy resources are enticing alternatives to our traditional reliance on the electric grid for all energy needs, while increasing the resilience of our energy system through additional flexibilities.”
Most of us understand electricity as something that comes from far and far away. Indeed, this is largely true, since most modernized electric power plants and generation facilities are located outside of major metropolitan areas, albeit only about 50 miles away from city centers. However, this wasn’t the case back in the early days of electric power.
When electricity was just beginning to take root in the everyday urban life of the 1880s, direct current (DC) developed by Thomas Edison was the standard in the United States. However, difficulties with transmission of the DC electricity over long distances required electric power generation plants to be located in or near city boundaries, consequently exacerbating environmental degradation of urban slums with smoke from fossil fuel combustion. In addressing DC’s drawbacks, alternating current (AC) developed by Nikola Tesla—which was much more effective and efficient at long-distance transmission—came out on top. Since then, AC became the go-to standard, and with it the centralization of electric power generation enabled by high voltage, long-distance AC transmission from outside of metropolitan areas.
AC model continues to proliferate in the contemporary electric power system. However, despite its advantages, it is not perfect. Furthermore, increasing risks to the transmission infrastructure due to natural disasters caused by climate change, such as wildfires, beg the question whether alternative options are available to help mitigate these risks. With the inventions of, and advances in, smaller electric power generation technologies such as photovoltaic (solar PV) panels coupled with energy storage systems that can be readily deployed inconspicuously in the urban environments, decentralized DC has been reemerging in the contemporary energy conversation.
Transportation electrification has been a key piece in the decentralization of electric power system, and therefore the global march toward distributed energy resources in the past two decades. The emergence of battery electric vehicles (BEV), plug-in hybrid electric vehicles (PHEV), and hybrid electric vehicles (HEVs) render energy storage in vehicles’ batteries for later use during peak demand hours, via vehicle-to-everything (V2X) technologies, closer to reality than it was ever before. This further allows communities to form and function within a microgrid (or alternatively a collection of mini and nano grids) that is operable as a subsystem to the larger grid and as a standalone system; increasing energy freedom and security.
As reliability concerns grow in parallel with frequent temporary service disconnections (e.g. public safety power shutoffs; PSPS), distributed energy resources are enticing alternatives to our traditional reliance on the electric grid for all energy needs, while increasing the resilience of our energy system through additional flexibilities. Decentralized generation and storage offers a host of benefits by feeding stored electricity to the grid when it is needed most, and provide continued service for critical end uses such as hospitals and households that require essential life-saving medical equipment to stay in operation.
While decentralized DC systems will grow in popularity, its disadvantages cannot be ignored. Much work remains in order to understand and successfully integrate distributed systems into the existing urban energy fabric. In the long term, we will most likely witness a growing relationship between AC and DC systems, as centralized and decentralized energy resources become a new norm. When this happens, the way we understand our buildings and cities will look dramatically different.